How Biochar enhances the long-term fertility of soil by improving the retention and exchange of water-soluble nutrients with plants.
By Austin Liu – March 2020
Preface and Background
I will address some background knowledge relevant to soil fertility and biochar that is not as widely known as it ought to be — the mechanism of nutrient retention and exchange in soil. I am specifically going to explain cation exchange capacity, CEC (cation is pronounced “cat ion”, not “cashun”) and anion exchange capacity, AEC, in a manner that is accessible to the layperson. CEC and AEC are two capacities which often show up in scientific literature and in news articles about soil science and soil fertility. High CEC and AEC are two of the quantifiable agronomic qualities of a fertile soil. Understanding what these are, why they are important, and how to to improve these capacities will help anyone who works with soil to reason soundly about soil amendments, including biochar, which I discuss toward the end of this article.
What Are Cations and Anions?
This is the layperson’s whirl-wind review of just enough chemistry to understand this article. Skip to the next section if you are familiar with this. Skip to the last paragraph of this section to simply read the definition of cations and anions.
All the stuff in the world is made of atoms, the units of the elements that make up all the matter in the world. Atoms have a nucleus which is positively charged, surrounded by electrons, which are negatively charged. In the case of these electrical charges, opposites attract. The designation of “positive” and “negative” are a reference to the number line in math, where positive numbers added to negative numbers of equal magnitude equal zero. An atom or molecule with an equal number of positive and negative charges has zero charge; it is electrically neutral. “Positive” and “negative” in this context do not designate good or bad. The assignment of positive and negative was arbitrarily assigned back in the 1700’s, when the science of electricity was in its infancy. Benjamin Franklin was one of the early contributors to the theory of electric charge who determined the convention of “positive” and “negative” electrical charge, back before it was known that electrons were the mobile charge carrier responsible for embodying negative electrical charges. According to his theory, it was believed that electrical current flows from positive to negative. In 1879, the American physicist Edwin Hall devised a clever experiment that discovered that the charges that actually flow in electric currents are the electrons, which carry negative charge, so physically speaking, electrical current actually flows from negative to positive. In 1909, the British physicist Ernest Rutherford discovered that the positive charges in an atom are all concentrated in the atomic nucleus. These two discoveries established the basic modern concept of the atom as we know it.
Atoms, in their whole, uncharged state, have an equal number of electrons and protons. When an atom has a net charge, such as having too many electrons or too few electrons to balance the charge from the protons in their nuclei, it is known as an ion. Cations are ions with a positive charge; they are missing one or more electrons, and therefore have a positive charge. Anions are ions with a negative charge; they have one or more extra electrons. Atoms aren’t the only things that can be ions. Molecules (atoms that are bonded to other atoms to form new substances) can also be ions if they have a net charge.
The Water-Solubility of Plant Nutrients and the Problem of Leaching
Water is the medium used by plants to take up nutrients from the soil; in order for plants to absorb and transport nutrients, these nutrients must be able to dissolve in water. However, this solubility in water also makes them prone to leaching out of the topsoil when it rains or when water is applied via irrigation. This is one of the reasons why tropical soils, which are thoroughly weathered by frequent rainfall, are such poor soils for agriculture. Everything in these tropical soils that can dissolve into water ends up dissolving and running off into the river. Eons of exposure to such a tropical climate results in heavily weathered soils leached of soluble nutrients.
If poorly managed, farm soils can experience the same fate. The way we have managed to farm on poor soils is to apply fertilizers that supply nutrients that the soil lacks. However, these fertilizers are themselves water soluble, and their leaching contributes to pollution in the form of algae blooms in the bodies of water where the run-off accumulates.
What then is the difference between a soil that can retain water soluble plant nutrients and one which cannot? The difference is each soil’s ion exchange capacity — cation exchange capacity for positive ions and anion exchange capacity for negative ions. Since the major water soluble plant nutrients are all ionic, the ability to capture and exchange ions results in the ability to capture and release water soluble nutrients. Some of the most important cation plant nutrients include:
- Potassium
- Magnesium
- Calcium
- Ammonium (as a source of reactive nitrogen)
- Iron
- Molybdenum
- Selenium
- Zinc
The major anion nutrients include:
- Nitrate (a source of reactive nitrogen)
- Phosphate
- Sulfate
- Chloride
[A note about nomenclature: Chemical names that end in -ate, -ite, and -ide usually designate anions, with various insoluble oxides being the general exception.]
These nutrient ions dissolve into water because the water molecule itself has a positively charged end and a negatively charged end that can attract these ions and pull them off of a solid into solution. Whereas the water molecule as a whole does not exhibit a net electrical charge, the electrons on a water molecule are not distributed evenly, resulting in one side exhibiting a permanent localized positive charge, and the opposite side exhibiting a permanent localized negative charge. This quality of having a positively charged side and a negatively charged side is known as being polar — having two poles (roughly speaking), each of which exhibit a local quality, in this case, electric charge.
Consider the shape of the water molecule. The chemical formula of water is H2O. Here is a written depiction you may be familiar with from high school chemistry:
Notice how the water molecule has a bend in it rather than being arranged in a straight line. This is because the oxygen atom has four outer orbitals arranged such that if the oxygen were in the center of a tetrahedron, the orbitals would point toward the four corners of the tetrahedron. Two of these orbitals are shared with hydrogen atoms, but two are each filled with a pair of electrons, represented above as the pairs of dots, each dot representing an electron. With the oxygen in the center of the tetrahedron, and hydrogens in the corners, the molecule has a bent shape. A three-dimensional representation of this arrangement can be seen here:
The side of the water molecule with the electron orbitals exhibits a local negative charge due to the presence of the electrons, while the side of the water molecule with the pair of hydrogen atoms (which are just protons, positively charged) exhibits a local positive charge.
The fact that water molecules exhibit these localized electrical charges is precisely how water dissolves soluble salts. In this context, ‘salt’ does not merely mean table salt (sodium chloride — sodium cations and chloride anions), although table salt is a great example. A salt, in this context, means any substance made of anions and cations, held together by the attraction of their opposite charges. For example, The chemical fertilizer ammonium nitrate is a salt made of ammonium cations and nitrate anions. Cations dissolve into water because their positive charge attracts the negatively charged portions of water molecules; water molecules then surround the cation and pull it off of the salt crystal. Anions dissolve into water because their negative charge attracts the positively charged portions of water molecules, which surround them and pull them into solution.
In order for dissolved ions to not run off as water drains out of the soil, the soil must have plenty of ion exchange sites — sites which can attract these dissolved ions well enough to capture them out of the water, but hold them loosely enough that the plant can get these ions off when they need them. For cations, these sites are known as cation exchange sites and for anions, these are known as anion exchange sites.
Cation Exchange Sites
Let us consider two types of cation exchange sites:
- Charged sites on the microstructure of various types of clay
- Oxygen-bearing functional groups found on soil organic carbon
Oxygen-bearing functional groups are much more relevant to biochar, so I will only briefly cover cation exchange in clay before examining the oxygen-bearing groups in detail.
Cation Exchange in Clay
Among the various mineral soil fractions, clay has the highest CEC in part because clay has tremendous microscopic surface area from all of the tiny and extremely thin flakes, but also because these flakes exhibit localized charges that attract ionic nutrients which are dissolved in the water. The surface area of a tiny clod of clay is many orders of magnitude higher than the equivalent grain of sand, akin to the combined surface area of both sides of all the pages of a large dictionary, which would amount to many hundreds of square feet, compared to the external surface area of the same book, which amounts to one or two square feet.
In the following micrograph, the layered microstructure of kaolinite clay can be seen. Other types of clay exhibit a similar flake microstructure.
The microstructure of clay consists of tiny flakes a few atoms thick, consisting of a repeating silicate crystal structure. These structures, like the water molecule, do not have their electrons evenly distributed, and have resulting localized charges along their surfaces and edges. These sites are responsible for the ability of clay to take up water, and to attract and weakly retain ionic nutrients.
Cation Exchange in Soil Organic Matter
Whereas clay has fairly good CEC compared to other soil constituents, the ability of clay to contribute CEC is dwarfed by the ability of soil organic carbon to do the same. To give you a sense of their relative CECs, see this scale illustrating the relative range of CEC exhibited by various types of soil and soil components:
In this scale, you can see that pure soil humus (soil organic matter) dominates the upper range, while vermiculites and smectites (which are types of clay) also exhibit fairly high CEC, but their CEC is significantly less than soil humus. Histosols (soils extremely rich in organic matter, from 20–30%) are in the same range as vermiculites and smectites. Finished compost is lower in the range, but as compost contributes to the formation of mature soil organic matter, and continues to break down into smaller particles, increasing in surface area, and continues to oxidize (explained below), the CEC continues to rise. This is consequential to horticulture and agriculture because soil organic matter is considerably easier to add to soil than changing its clay content, while having a greater influence on its CEC in the long run. Adding soil organic carbon also has the climate benefit of storing carbon in the soil. Additionally, the presence of excessive clay in the soil can be problematic, since clay soil can be extremely dense or even become impenetrable to plant roots and impermeable to water. In light of what I explained about how water dissolves ionic nutrients, lets see why soil organic matter has such high CEC.
Soil organic matter and humus consists of the decomposed residues of living organisms and their tissues, and its cation exchange qualities come from the abundance of oxygen-bearing functional chemical structures bonded onto its carbon structure. These functional structures are known as moieties in organic chemistry parlance. Remember, from our prior discussion of the structure of the water molecule, that oxygen has a pair of filled electron orbitals that give a kink to structures that contain oxygen, as well as giving that area a localized negative charge which mildly attract positively charged ions (cations). That is how simple structures such as the hydroxyl (an oxygen and a hydrogen) and carbonyl (a single oxygen double-bonded to a carbon) groups contribute to CEC, as well as to water retention, where the localized negative charge attracts the positive end of water molecules.
When a carbonyl and a hydroxyl group are bonded to the same carbon, that structure is called a carboxyl group or moiety, which carries two oxygen atoms. The carboxyl moiety is the most important structure that confers cation exchange capacity in soil organic matter. The carboxyl moiety is the structure responsible for the acidic quality of weak organic acids such as lactic acid, citric acid, and acetic acid (vinegar).
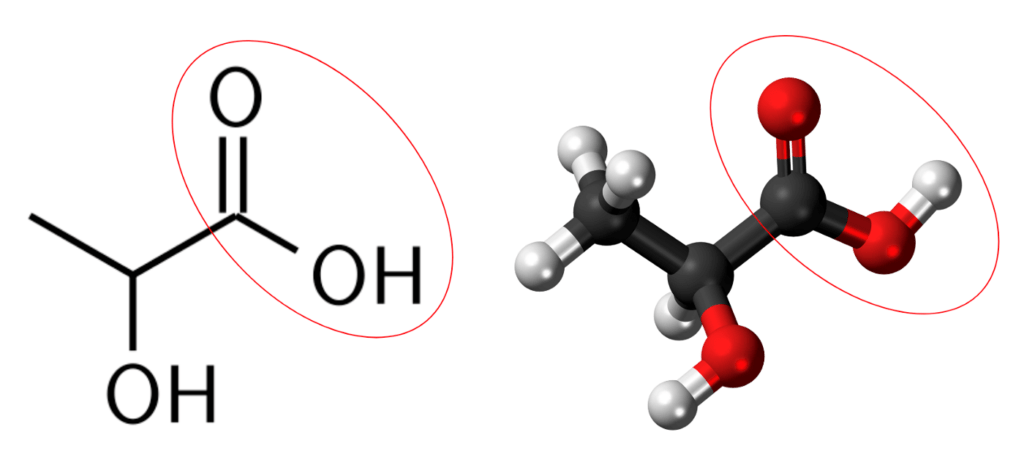
Acids release hydrogen cations (H+) into water, leaving the acid’s negatively charged anion, which is then free to attract and hold on to other cations, such as the nutrient ions plants need.
In the graphic shown above, the white ball (hydrogen) bonded to the red ball (oxygen) is the one that gets released into water as a hydrogen cation. The portion that is left behind is the acid’s anion. Remember that weak acids differ from strong acids in that they do not instantly and completely release their hydrogen ions into the water like strong acids do. Strong acids hardly hold on to their cations at all, whereas weak acids at least have a weak grip on their cations. This weak grip enables the carboxyl group, after it has released its hydrogen cation and become a carboxylate anion, to attract nutrient cations and weakly hold on to them.
How Plants Extract Nutrients from Cation Exchange Sites
How then does a plant root get the cation nutrient off of the carboxylate cation exchange site or even off of clay? The plant metabolizes some of its own carbohydrates, and releases the carbon dioxide through its roots into the soil, where it comes in contact with water and becomes carbonic acid. Carbonic acid then releases hydrogen cations into the water, and these hydrogen cations displace nutrient cations off of the carboxylate group, enabling the nutrient to diffuse up into the root. Alternatively, plants can also release H+ ions directly into the rhizosphere to mildly acidify the soil to release cation nutrients from cation exchange sites.
Weak Organic Acids, Biochar, and Compost
In light of the role of weak organic acids, how could one boost the CEC of one’s biochar? By co-composting the biochar — that is, to send it through the composting process. Aerated compost piles are hotbeds of aerobic bacterial fermentation by a diverse mixture of bacterial decomposers. Bacterial fermentation tends to produce weak organic acids, many of which react with and become incorporated into the coating that forms on biochar when it goes through composting. Many familiar bacteria fermented foods serve as examples of this: lacto-fermented pickles and sauerkraut (which contain lactic acid), vinegar (which contains acetic acid), and kombucha (which contains lactic, acetic, gluconic, and glucuronic acids). At the same time, decomposing organic matter often produces ammonia and amine-containing substances from all the nitrogen-bearing materials, while many alkaline mineral nutrients are released in the process of decomposition; since these substances are basic, the overall compost mixture does not necessarily become more and more acidic as composting progresses. Much of the ammonia that would otherwise be emitted from compost reacts with biochar and becomes bonded to its surface, contributing to anion exchange capacity (discussed below).
Anion Exchange Sites
Anion exchange capacity is particularly important for retaining nitrate and phosphate, two of the most important anion plant nutrients, keeping them in plant-available form, and protecting these from leaching out of the soil and polluting groundwater and rivers.
Just as cation exchange sites use their negative charge to attract and hold on to positively charged cation nutrients, anion exchange sites exhibit a positive charge to attract and hold on to negatively charged anion nutrients. Since much of the scientific principles involved in anion exchange are the same as cation exchange, and were explained above, this section will focus on the differences.
Anion Exchange in Clay
Most types of clay (smectite, vermiculite, montmorillonite, bentonite) expose localized negative charges on their microstructure, which make them great for cation exchange, but they are typically poor at anion exchange. The exception to this rule is kaolinite clay, which is great at anion exchange but poor at cation exchange because its microstructure exposes localized positive charges instead. This can be seen in the CEC distribution scale shown earlier in the article. Kaolinite is at the very bottom, with hardly any CEC.
Intense and prolonged weathering of clay minerals can break down clays which are high in CEC and form kaolinite clays, and eventually, iron and aluminum oxide clays, which are also characterized by low CEC but high AEC.
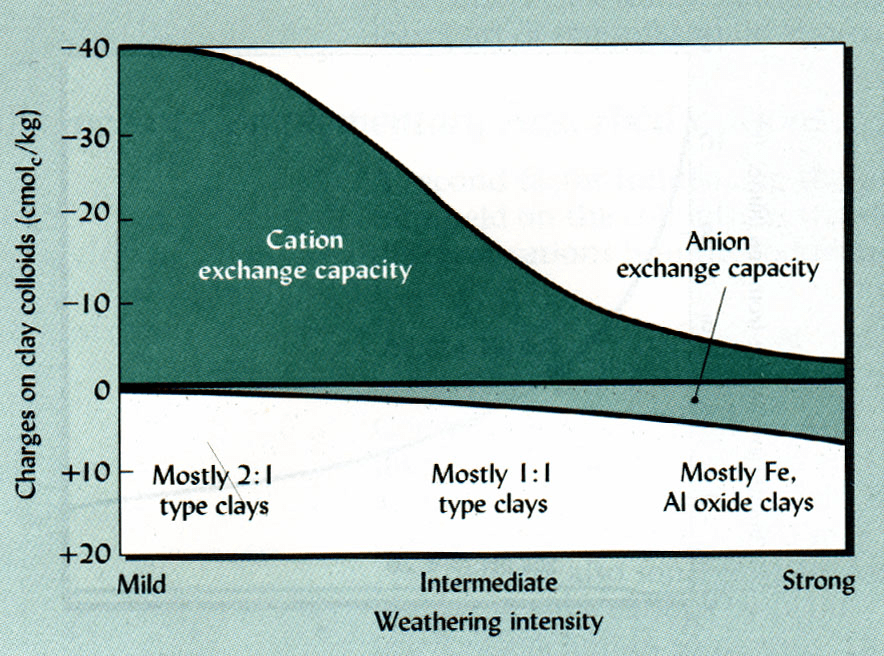
Anion Exchange in Soil Organic Matter and Co-Composted Biochar
Anion exchange in soil organic matter is carried out by positively charged functional groups, just as cation exchange is carried out by negatively charged functional groups. Let us consider two examples found in co-composted biochar.
When biochar is composted, it reacts with and traps a stunning amount of ammonia, which is produced as nitrogen-containing materials in the compost decompose. In 2019, a paper by the Cornell University soil sciences group (with Prof. Johannes Lehmann and Dr. Tianran Sun) reported that more than half of this ammonia doesn’t merely adsorb onto the surface of the char, but actually reacts with it, forming amine and other nitrogen containing moieties. The amine moiety looks like the circled part of the molecules shown here:
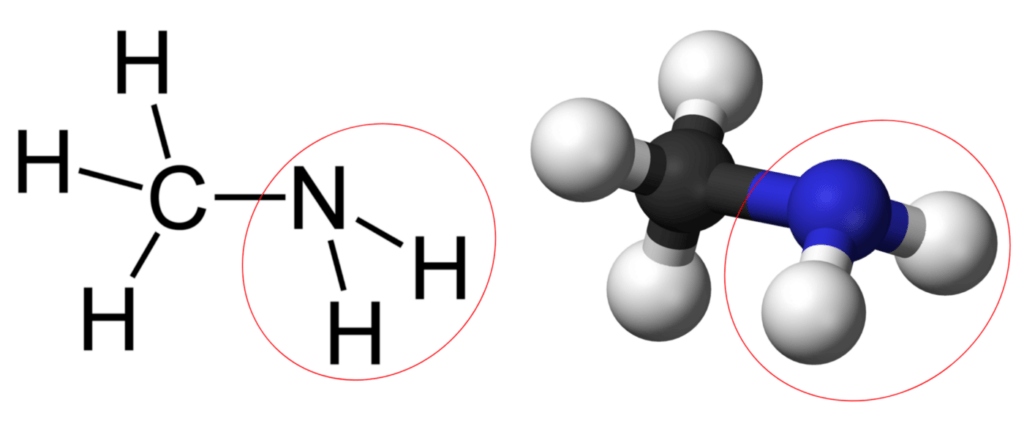
Observe that the amine has a kink in it as well. Similar to oxygen, the nitrogen in the amine group has a pair of electrons in a filled orbital sticking out where the kink is (whereas oxygen has two filled orbitals). However, the electron cloud on the amine structure can sometimes pull hydrogen off of water as an cation, releasing the remaining portion of the water, OH-, as a hydroxide ion. This production of OH- ions is what makes the amine group a weak base, the counterpart to weak organic acids. Once it has pulled a hydrogen cation to itself, the structure is positively charged, and can attract and retain anions. In the same way that a weak acid moiety can release its hydrogen cation to become negatively charged, and use this charge to attract and hold on to cation nutrients in its place, a weak base moiety can pull a hydrogen cation (or other cations besides hydrogen) to itself to become positively charged, and use this positive charge to attract and hold on to anion nutrients such as nitrates.
In fact, nitrate retention in co-composted biochar appears to be one of the mechanisms by which it confers benefits to plants. This ability of biochar to capture and react with ammonia to form anion-exchange sites would explain a curious observation from this paper from 2015, added emphasis in bold:
“Electro-ultra filtration and sequential biochar-particle washing revealed that co-composted biochar was nutrient-enriched, particularly with the anions nitrate and phosphate. The captured nitrate in BCcomp was (1) only partly detectable with standard methods, (2) largely protected against leaching, (3) partly plant-available and (4) did not stimulate N2O emissions.”
Plant growth improvement mediated by nitrate capture in co-composted biochar: https://www.nature.com/articles/srep11080#Sec10
I venture to speculate, in light of the more recent paper on biochar’s ability to bond to ammonia, that the rich amount of the anions nitrate and phosphate that were protected against leaching were held in the biochar by means of anion exchange sites from the amine groups that form when ammonia covalently bonds to the carbon in the biochar during composting, a phenomenon not reported in the scientific literature until 2019. Speculating from the 2019 report about ammonia reacting with and bonding to char, the nitrogen taken up by the char that is no longer plant-available, as reported in the quote, may be the fraction that has covalently bonded to the char.
Since anions are attracted to positive charges on structures attached to the surface of biochar, another plausible anion exchange site should be noted. The carboxyl moiety, responsible for cation exchange, usually gives up its hydrogen (H+) and retains another cation with a 1+ charge when it carries out cation exchange. When it does this, there is no net charge on the structure. However, if it instead retains a cation with a 2+ charge, such as calcium (Ca2+) or magnesium (Mg2+), there is a net 1+ charge left, so this positive charge could then attract and retain anions.
How Plants Extract Nutrients from Anion Exchange Sites
Plants do not merely release carbon dioxide into the soil through their roots to release cation and anion nutrients using the hydrogen and carbonate ions from the carbonic acid. Plants are also able to exude directly carbonates and bicarbonates as well as hydrogen cations directly into the soil to manipulate the local pH around the root to release nutrients on-demand. Just as the hydrogen cation can displace a cation nutrient off of a cation exchange site, making it available to be absorbed into the root, the carbonate anion can displace an anion nutrient off of an anion exchange site, making it available to be absorbed into the root.
What Improved Nutrient Exchange Affords
When the CEC and AEC of soil is significantly improved, such as by the addition of co-composted biochar, two effects related to nutrient exchange will be observed:
1 – Greater Fertilizer Utilization Efficiency
If you do use fertilizers on your crops, expect the utilization efficiency of the fertilizer to be much improved after amending the soil with co-composted biochar and enriching your soil organic carbon by regenerative farming practices; more of the dissolved fertilizer ions would be caught by ion exchange sites to be made available to your plants, and less of it would be wasted. This is a really big deal; the state of fertilizer utilization efficiency on American farms is dismal according to this remark from the Better Crops agricultural journal, quote:
“… research on farm fields in the U.S. and Asia shows that apparent single-year recovery efficiency for fertilizer N is usually below 50% and frequently below 40%, illustrating significant opportunity for improvement.”
State of Fertilizer Utilization Efficiency, Better Crops (2003)
The 2013 soil science documentary Symphony of the Soil by Debora Koons Garcia (highly recommended for anyone interested in soil) had an interview with Dr. David R. Montgomery, the author of Dirt: The Erosion of Civilizations, who asserted that the fertilizer utilization efficiency of the typical American farm soil was 30%. In the same documentary, Dr. Laurie Drinkwater, of the Department of Horticulture at Cornell University, remarked that in vegetable systems, efficiency can be as low as 20%; as much as 80% of the fertilizer is lost. This has stunning implications; this would mean that more than half of the fertilizer applied to the average American farm goes to waste, whether by off-gassing or by run-off, incurring unnecessary pollution in its production and in the waters that receive the run-off, let alone the wasted money involved in purchasing and transporting this much fertilizer. The production of fertilizer is itself extremely damaging to the environment, incurring massive methane emissions far worse than what the industry self reports. Since the utilization efficiency is so critically dependent on retaining nutrients and effective exchange of nutrients with plants, boosting the CEC and AEC of your soil will give you much more effective use of any fertilizers you do apply.
2 – Cleaner Run-Off
Having higher CEC and AEC in your soil does not stop water from draining from your land , but the water that does drain from the land will be much cleaner, as the dissolved nutrient ions that come off of poor soil would be much reduced in water draining off of soil with strong nutrient capture and retention. In addition, the chemical mechanisms involved in CEC and AEC in soil organic carbon are also associated with improved water retention. From the perspective of pollution abatement — namely, the reduction of fertilizer pollution, algae blooms, nitrate pollution in drinking water, and the stinking dead zones that follow — improving the CEC and AEC of agricultural land is one of the most impactful measures that can be taken to counter fertilizer pollution.
Summary and Conclusion
The effective fertility of your soil depends on so many factors, but the end result can be fairly well described in just a handful of quantifiable metrics, such as soil aggregate stability, water holding capacity, and nutrient exchange capacity — specifically, cation exchange capacity and anion exchange capacity, CEC and AEC. CEC and AEC are particularly important because they impact the very mechanism by which soil retains nutrients against leaching and makes them available for plants. Water soluble ionic nutrients are protected against leaching by being attracted and held at chemical functional groups with the opposite charge. Plants release these ionic nutrients by exuding hydrogen or carbonate ions, according to what they need, to displace the nutrients from these sites. Whereas CEC and AEC are influenced by the clay content of your soil, they can be significantly improved by the addition of co-composted biochar, and by regenerative farming practices which foster the accumulation and maturation of soil organic carbon. Co-composting biochar increases the CEC by the addition of weak organic acid groups to the surface of the char via the organic coating that adheres to the char during composting. In addition, biochar reacts with and covalently bonds with ammonia in the compost pile, reducing ammonia emissions while increasing its AEC. The remarkable levels of nitrate and phosphate anions retained on co-composted biochar which are protected against leaching support this inference.
By this means, the poor fertilizer utilization efficiency of our agricultural lands and even our home lawns and gardens can be improved, reducing the waste of fertilizer and abating the pollution caused by fertilizer runoff.
Post-script: Fertilizer vs. Fertility
From all this talk of fertilizer utilization efficiency, you may come away from this with the impression that co-composted biochar is about improving the use of fertilizer. Whereas it can do that, that is not the point of our work with biochar. Fertilizer utilization efficiency may be more generally thought of as nutrient utilization efficiency. Modern industrial agriculture is dependent on fertilizer precisely because the use of fertilizer, along with the use of herbicides and other agrochemicals, harms the soil microbiome’s ability to confer fertility to the soil. It is precisely because of the infertility of badly farmed soil that fertilizers are needed to produce any crops. If you are not familiar with the problem, I recommend that you watch the fantastic soil documentary Symphony of the Soil, which covers this topic in a very accessible and compelling manner. The objective of everything of which I am informing you is not to promote the better use of fertilizer; the objective is to understand how to cultivate long term soil fertility—the supporting and propagating of life and its abundance. The ideal would be healthy and fertile soil, maintained or even improved by our stewardship of it, while we merely take a cut of its abundance to supply our food, while returning our waste products to the land to prevent its impoverishment.
Historically, access to reactive nitrogen has been a limiting factor for the growth of our food crops. (The nitrogen in the atmosphere is abundant but is effectively inert, and is useless as a source of nitrogen for plant life.) The development of synthetic methods for producing reactive nitrogen in the early 1900’s resulted in a glut of reactive nitrogen; any failure on the part of nutrient retention and exchange—the elements of fertilizer utilization efficiency—was hidden from us because we could simply supply more fertilizer. This only further harmed the soil, while causing fertilizer run-off pollution, air pollution, and other problems.
Organic farming alone does not solve the problem; if one farms in a conventional manner, and simply substitutes organic inputs for conventional inputs, the soil is hardly helped. It is necessary to go beyond mere organic farming; it is necessary to farm regeneratively, to regenerate the soil’s fertility. Since reactive nitrogen is so energetically expensive to produce and is difficult to come by using natural means, the first step in weening one’s land off of fertilizer dependence is to reduce the amount that is needed in the first place. This is accomplished by radically improving nutrient retention and exchange, which improves fertilizer utilization efficiency. The second step is to boost the natural sources of reactive nitrogen in the soil, whose precious output would be wasted if the soil cannot retain and exchange nutrients efficiently. We’ll look at natural sources of soil reactive nitrogen in a future installment of this series.